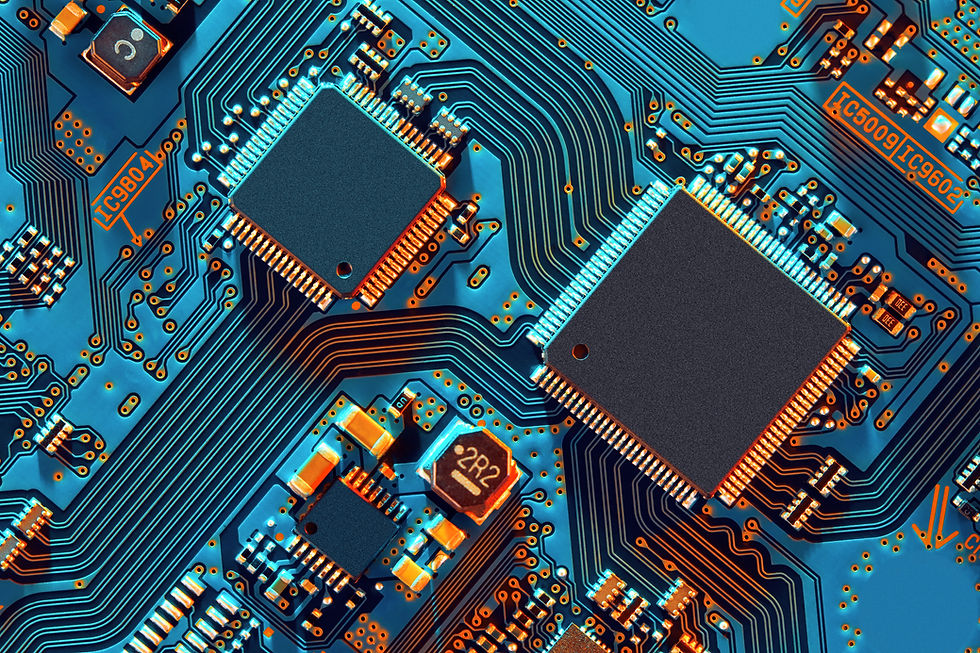
Executive Summary
Post-Quantum Cryptography (PQC) is a critical field addressing the security threats posed by quantum computers to current encryption methods. Quantum computers could break widely used cryptographic algorithms like RSA and ECC. PQC aims to develop quantum-resistant algorithms to ensure long-term data security.
Key points:
Quantum Threat: Quantum computers can potentially break classical cryptographic algorithms.
PQC Development: NIST has announced the first standardized PQC algorithms.
Organizational Impact: Organizations need to prepare for the transition to PQC.
Sector-Specific Considerations: Nonprofits, SMBs, and corporations face unique challenges.
Case Studies: Incidents like the YubiKey side-channel attack highlight the need for robust cryptography.
Recommendations: Organizations should assess, educate, and plan for PQC implementation.
Open-Source Support: Initiatives are aiding PQC development and adoption.
This document provides a comprehensive overview of PQC, its significance, current standards, sector impacts, case studies, and steps for organizations to incorporate quantum-safe cryptography.
Table of Contents
Introduction
Understanding Post-Quantum Cryptography
The Significance of Post-Quantum Cryptography
Practical Implications of PQC
Current State of PQC Standards
PQC Timeline and Milestones
Latest and Emerging Trends in PQC
Quantum-Safe Alternatives
Steps for Organizations to Incorporate PQC
Challenges and Limitations of PQC
Industry-Specific PQC Considerations
11.1 Nonprofits
11.2 Small and Medium-Sized Businesses (SMBs)
11.3 Corporations
Global Perspectives on PQC
Security Incidents and Case Studies
13.1 Case Study 1: Superfish Incident (2015)
13.2 Case Study 2: YubiKey Side-Channel Attack (2024)
13.3 Additional Case Studies
PQC Algorithms and Standards
Implementation Challenges
Recommendations for Organizations
Open-Source Organizations and Resources
Conclusion
Further Reading
Glossary of PQC Terms
Frequently Asked Questions
Citations
1. Introduction
As we stand on the brink of a new era in computing, the emergence of quantum computers poses a significant challenge to traditional cryptography. The rise of these powerful machines threatens to break many of the encryption schemes that have kept our data secure for decades. In response, the field of Post-Quantum Cryptography (PQC) has emerged, aiming to develop cryptographic algorithms that can withstand the capabilities of quantum computers.
2. Understanding Post-Quantum Cryptography
Post-Quantum Cryptography refers to cryptographic algorithms designed to be secure against both classical and quantum computer attacks. Quantum computers operate on the principles of quantum mechanics, enabling them to solve certain mathematical problems exponentially faster than classical computers. This capability could render widely used cryptographic algorithms, such as RSA and ECC (Elliptic Curve Cryptography), vulnerable.
PQC focuses on developing algorithms based on mathematical problems believed to be resistant to quantum attacks, such as:
Lattice-based cryptography
Hash-based signatures
Multivariate polynomial equations
Code-based schemes
3. The Significance of Post-Quantum Cryptography
The significance of PQC cannot be overstated. With the advent of quantum computing, organizations must prepare for a paradigm shift in security. A successful quantum attack could lead to:
Data Breaches: Sensitive data encrypted with current algorithms could be exposed.
Loss of Trust: The integrity of digital communications could be compromised.
Financial Impacts: Data breaches could lead to significant financial losses.
National Security Risks: Classified information and critical infrastructure could be vulnerable.
Long-term Data Exposure: Data intercepted today could be decrypted in the future ("harvest now, decrypt later" attacks).
4. Practical Implications of PQC
The transition to PQC will have far-reaching effects:
Infrastructure Upgrades: Significant hardware and software changes may be required.
Increased Computational Overhead: PQC algorithms may require more resources.
Data Protection Lifecycle: Long-term data storage must be re-evaluated.
Compliance and Regulation: New standards will emerge, requiring adaptation.
Supply Chain Security: Ensuring vendors and partners transition to PQC is essential.
Key Management Complexity: Larger key sizes will complicate key management.
Network Bandwidth: Larger key sizes may increase network traffic.
User Experience: Transition may impact user experience due to processing times.
Legacy System Compatibility: Ensuring compatibility will be a significant challenge.
Education and Training: Investing in workforce education on PQC is crucial.
5. Current State of PQC Standards
The National Institute of Standards and Technology (NIST) is leading efforts to standardize PQC algorithms. As of August 2024, NIST has released the following finalized standards:
FIPS 203: Module-Lattice-Based Key-Encapsulation Mechanism (ML-KEM), based on CRYSTALS-Kyber.
FIPS 204: Module-Lattice-Based Digital Signature Algorithm (ML-DSA), based on CRYSTALS-Dilithium.
FIPS 205: Stateless Hash-Based Digital Signature Algorithm (SLH-DSA), based on SPHINCS+.
A draft standard for FALCON is expected by late 2024.
6. PQC Timeline and Milestones
2015: NIST initiates the PQC standardization process.
2022: NIST announces first group of PQC algorithms for standardization.
2024 (August): NIST releases first three finalized PQC standards.
2025-2030: Projected widespread adoption of PQC.
2030+: Potential arrival of cryptographically relevant quantum computers.
Organizations should aim to be "crypto-agile" by 2025, capable of swiftly transitioning to PQC algorithms as needed.
7. Latest and Emerging Trends in PQC
Emerging trends include:
Hybrid Cryptography: Combining traditional and PQC algorithms.
Standardization Efforts: Global organizations working on PQC standards.
Increased Research Funding: Governments and private sectors investing in PQC research.
Implementation and Testing: Real-world applications are being tested.
Crypto-Agility: Developing systems that can quickly adapt to new algorithms.
8. Quantum-Safe Alternatives
While PQC is the primary focus, other approaches include:
Quantum Key Distribution (QKD): Uses quantum mechanics for secure key exchange.
Physical Unclonable Functions (PUFs): Hardware-based security method.
One-Time Pad: Theoretically unbreakable if used correctly.
Noise-Based Cryptography: Utilizes physical noise for encryption.
Quantum-Resistant Blockchain: Securing blockchain technologies with PQC algorithms.
9. Steps for Organizations to Incorporate PQC
Assess Current Cryptographic Infrastructure.
Stay Informed about PQC developments.
Plan for Migration with a strategic timeline.
Invest in Training for IT staff and decision-makers.
Implement Crypto-Agility in systems.
Conduct Pilot Projects for testing PQC algorithms.
Update Security Policies and Procedures.
Engage with Vendors and Partners on PQC readiness.
Monitor and Adapt to the evolving quantum threat landscape.
Consider Hybrid Approaches during transition.
Perform Risk Assessments regularly.
10. Challenges and Limitations of PQC
Organizations face several challenges:
Performance Overhead: PQC algorithms may require more computational resources.
Key and Signature Sizes: Larger sizes impact storage and transmission.
Compatibility: Ensuring interoperability during the transition.
Standardization Process: Ongoing and may change.
Quantum Threat Timeline: Uncertain timeline complicates planning.
Skills Gap: Shortage of expertise in PQC.
Implementation Errors: Risk of vulnerabilities due to errors.
Side-Channel Attacks: Some PQC algorithms may be susceptible.
Quantum-Safe Random Number Generation: Crucial for security.
Cost of Transition: Significant investment required.
11. Industry-Specific PQC Considerations
11.1 Nonprofits
Nonprofits often handle sensitive donor information and may engage in confidential communications related to their missions. The impact of PQC on nonprofits includes:
Data Protection: Safeguarding donor information against future quantum attacks.
Resource Allocation: Challenges in allocating resources for PQC implementation.
Compliance: May need to comply with PQC standards to maintain partnerships and funding.
11.2 Small and Medium-Sized Businesses (SMBs)
SMBs face unique challenges and opportunities:
Competitive Advantage: Early adoption could provide a competitive edge.
Cost Considerations: Significant investment may strain budgets.
Supply Chain Security: Ensuring suppliers and partners are PQC-compliant.
11.3 Corporations
Large corporations face complex challenges:
Legacy Systems: Updating extensive systems is time-consuming and expensive.
Global Compliance: Navigating varying PQC standards internationally.
Research and Development: Investing in developing PQC solutions.
12. Global Perspectives on PQC
Approaches to PQC vary globally:
United States: NIST leading standardization; significant government funding.
European Union: ETSI working on PQC standards; focus on GDPR compliance.
China: Developing its own PQC algorithms; significant investment in research.
Other Regions: Japan, Australia, Canada, Israel, and others are actively engaged in PQC research and implementation.
13. Security Incidents and Case Studies
13.1 Case Study 1: Superfish Incident (2015)
Lenovo pre-installed Superfish adware on consumer laptops, compromising SSL/TLS connections by installing a self-signed root certificate. This allowed potential man-in-the-middle attacks, demonstrating the need for strong cryptographic practices [2].
13.2 Case Study 2: YubiKey Side-Channel Attack (2024)
A side-channel vulnerability, named EUCLEAK, was discovered in older YubiKey models, potentially allowing attackers to clone devices [1][3]. Key points:
Vulnerability: Exists in the Infineon cryptographic library.
Exploitation: Requires physical access and specialized equipment.
Significance: Highlights the importance of updating cryptographic implementations.
13.3 Additional Case Studies
IBM's PQC Implementation: Integrated PQC algorithms into cloud services.
Google's PQC Experiment: Tested PQC in Chrome browser.
Volkswagen's Quantum-Safe Signatures: Exploring quantum-resistant digital signatures for vehicles.
Microsoft's PQC in Azure: Implemented PQC protections in IoT security solutions.
14. PQC Algorithms and Standards
NIST is leading efforts to standardize PQC algorithms. Promising candidates include:
Lattice-based: CRYSTALS-Kyber for key encapsulation.
Hash-based: SPHINCS+ for digital signatures.
Code-based: Classic McEliece for key encapsulation.
Multivariate: Rainbow for digital signatures.
15. Implementation Challenges
Organizations face several challenges when implementing PQC:
Performance: Some algorithms require more computational resources.
Compatibility: Ensuring solutions work with existing systems.
Standardization: Avoiding rework by waiting for final standards.
Crypto-Agility: Designing systems to switch between algorithms easily.
16. Recommendations for Organizations
Assessment: Evaluate current cryptographic practices.
Education: Train staff about PQC and its implications.
Phased Approach: Implement PQC in stages.
Hybrid Solutions: Use hybrid classical-PQC solutions during transition.
Stay Informed: Keep up-to-date with standards and best practices.
17. Open-Source Organizations and Resources
Open Quantum Safe (OQS): Supports development of quantum-resistant cryptography.
PQClean: Provides clean, portable implementations of PQC algorithms.
PQCgenKAT: Generates test files for PQC algorithm validation.
18. Conclusion
Post-Quantum Cryptography is crucial for maintaining long-term data security across all sectors. While the transition presents challenges, particularly for resource-constrained organizations, the potential risks of not adopting PQC far outweigh the implementation costs. Incidents like the YubiKey side-channel attack emphasize the need for robust cryptographic solutions.
As we move forward, collaboration between governments, industry, academia, and the open-source community is essential. By working together and staying informed about the latest developments in PQC, we can ensure the continued security and privacy of our digital world in the face of advancing quantum technologies.
The time to act is now.
19. Further Reading
NIST Post-Quantum Cryptography Standardization: NIST PQC
"Post-Quantum Cryptography" by Daniel J. Bernstein et al.
European Telecommunications Standards Institute (ETSI) Quantum-Safe Cryptography: ETSI
IBM's Quantum-Safe Cryptography: IBM Quantum-Safe
Cloud Security Alliance's Quantum-Safe Security Working Group
20. Glossary of PQC Terms
Quantum Computer: A computer using quantum-mechanical phenomena.
Cryptographically Relevant Quantum Computer: Capable of breaking current cryptographic systems.
Shor's Algorithm: Quantum algorithm for factoring large numbers.
Grover's Algorithm: Quantum algorithm for searching unsorted databases.
Lattice-based Cryptography: Based on lattice problems.
Hash-based Signatures: Rely on cryptographic hash functions.
Crypto-Agility: Ability to switch cryptographic algorithms swiftly.
Quantum Key Distribution (QKD): Secure communication method using quantum mechanics.
Hybrid Cryptography: Combines classical and PQC algorithms.
21. Frequently Asked Questions
When will quantum computers be able to break current encryption?
Estimates range from 5 to 15 years. Preparing now is crucial.
Will PQC completely replace current cryptographic systems?
PQC will likely be used alongside current systems initially.
Are PQC algorithms slower than current ones?
Some are slower or require more resources; ongoing research aims to improve efficiency.
How can organizations start preparing for PQC?
Assess cryptographic infrastructure, stay informed, and plan for migration.
Are there PQC solutions available now?
Yes, but widespread standardization is still in progress.
22. Citations
[1] The Verge [2] SC World [3] Infosecurity Magazine [4] CSO Online [5] Yubico Security Advisory [6] Ars Technica
About the Author
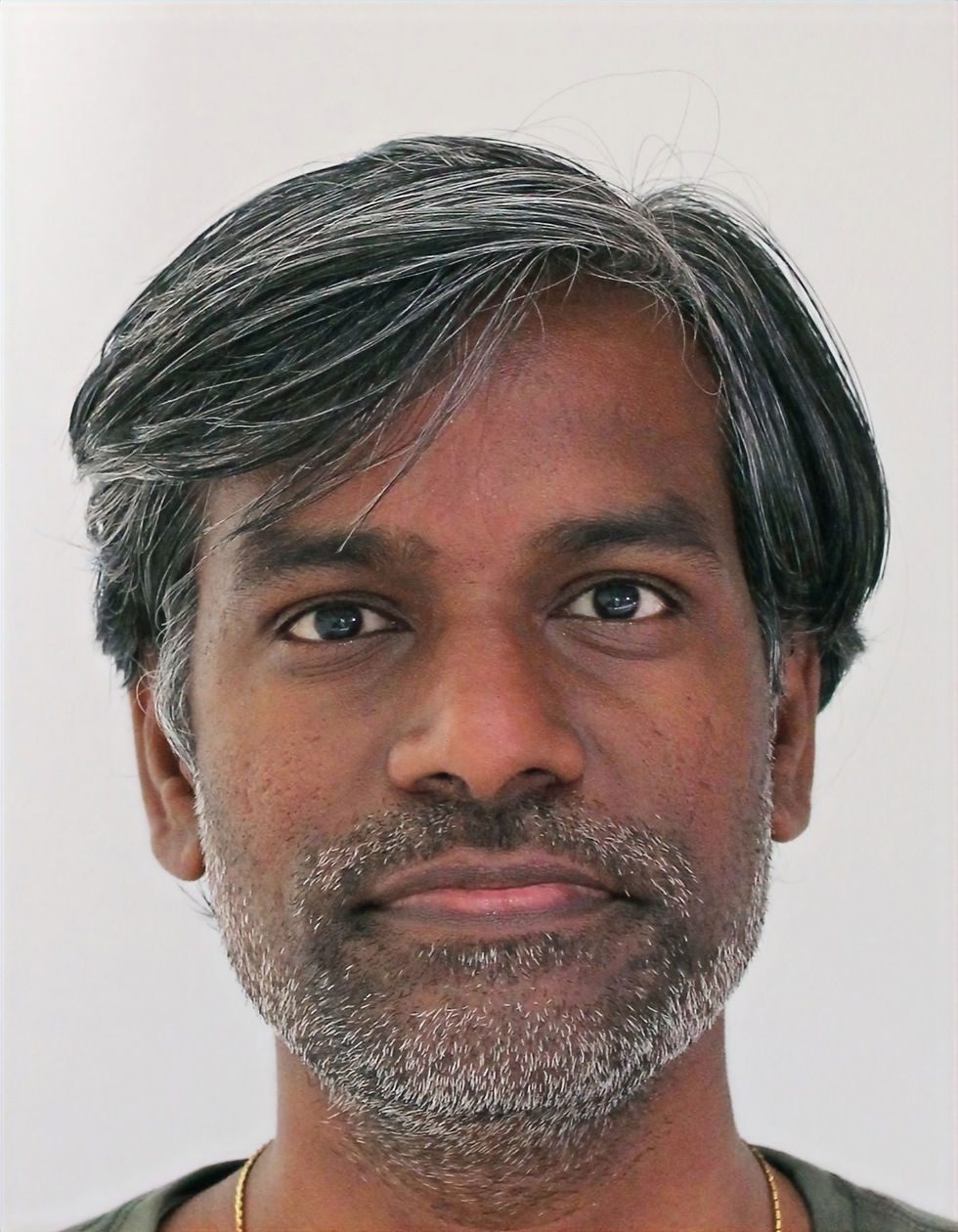
Santhosh Kumar Edukulla is the Vice President of Research & Security Engineering at CyberSecurity NonProfit (CSNP). With over 22 years of experience in software engineering and cybersecurity, Santhosh brings a wealth of knowledge and expertise to the field of post-quantum cryptography.
Prior to joining CSNP, Santhosh held senior positions at industry giants including Google and Amazon, where he contributed to cutting-edge security initiatives. His innovative work has resulted in 9 filed patents, demonstrating his commitment to advancing the field of cybersecurity.
Santhosh's research interests focus on the intersection of quantum computing and cryptography, with a particular emphasis on developing practical, quantum-resistant security solutions. He is a strong advocate for open-source development and actively contributes to various community-driven cybersecurity projects.
As a recognized thought leader in the industry, Santhosh frequently speaks at international conferences and has authored numerous articles on emerging cybersecurity trends. His unique blend of technical expertise and strategic vision positions him at the forefront of preparing organizations for the post-quantum era.
Santhosh holds a Master's degree in Computer Science with a specialization in Cryptography from [University Name]. He is also a Certified Information Systems Security Professional (CISSP) and a member of the International Association for Cryptologic Research (IACR).
Comments